DOWNLOAD YOUR FREE EBOOK
Learn how the unique shapes players make in motion can unlock new levels of speed in the game.
Level Up Coaching Techniques: Mastering Movement for Maximum Velocity
Upgrade your speed coaching with the free ALTIS Shapes for Speed eBook. This concise guide, drawn from our NSCA Accredited Need for Speed course, reveals how understanding the 'shapes' of acceleration and maximum velocity can dramatically enhance speed in the game.
WHAT YOU'LL LEARN
- The foundational concept of "shapes" in athlete movement, essential for speed and agility development.
- How to analyze athletes' sprinting techniques and identify effective vs. ineffective movement patterns.
- The significance of the ALTIS Coaching Framework and its application in coaching for speed improvement.
- Strategies for incorporating the understanding of movement shapes into practical coaching methods.
- The importance of differentiating between the intention and outcome of movements to enhance athlete performance.
- Techniques to improve coordination and efficiency in athletes, leading to better performance on the field.
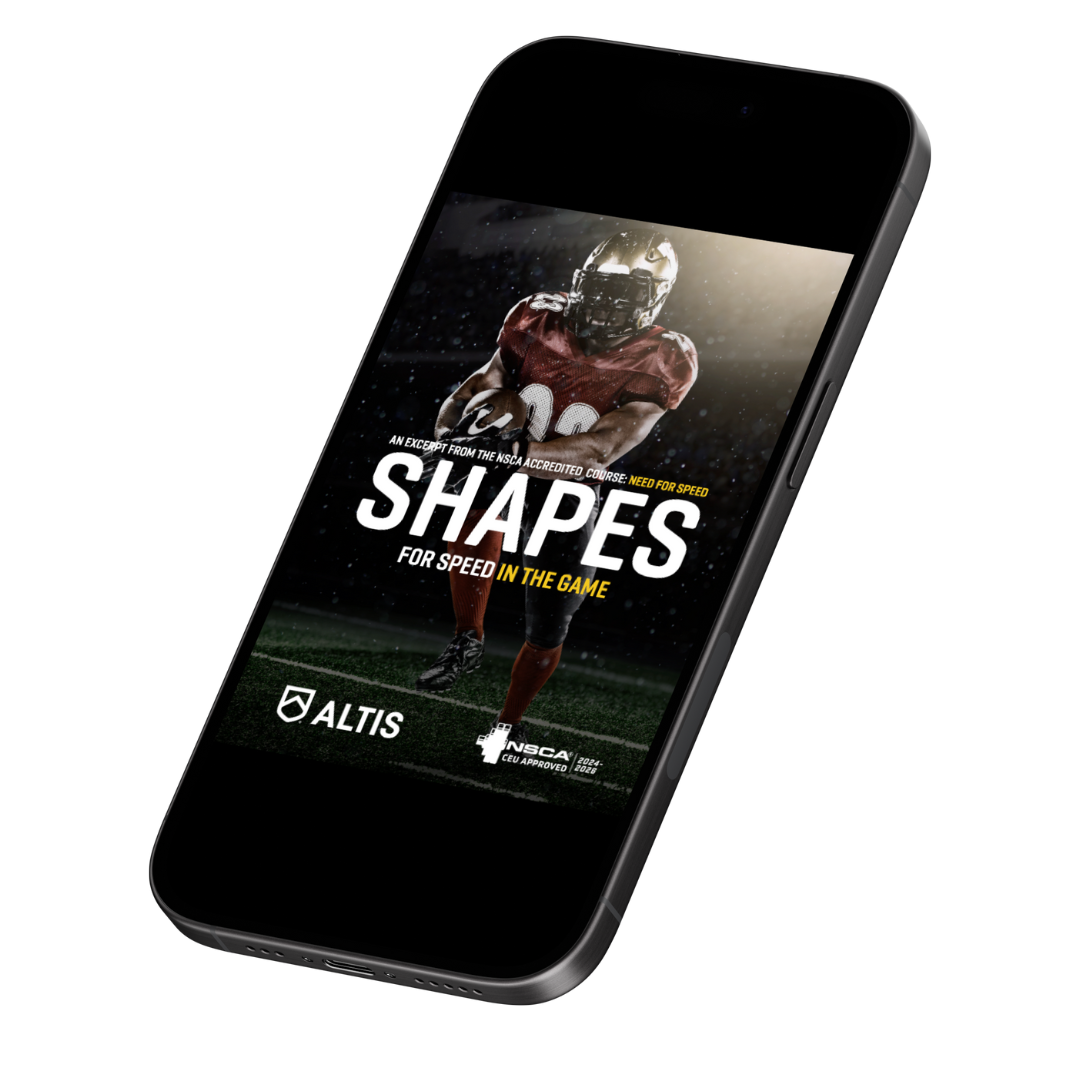
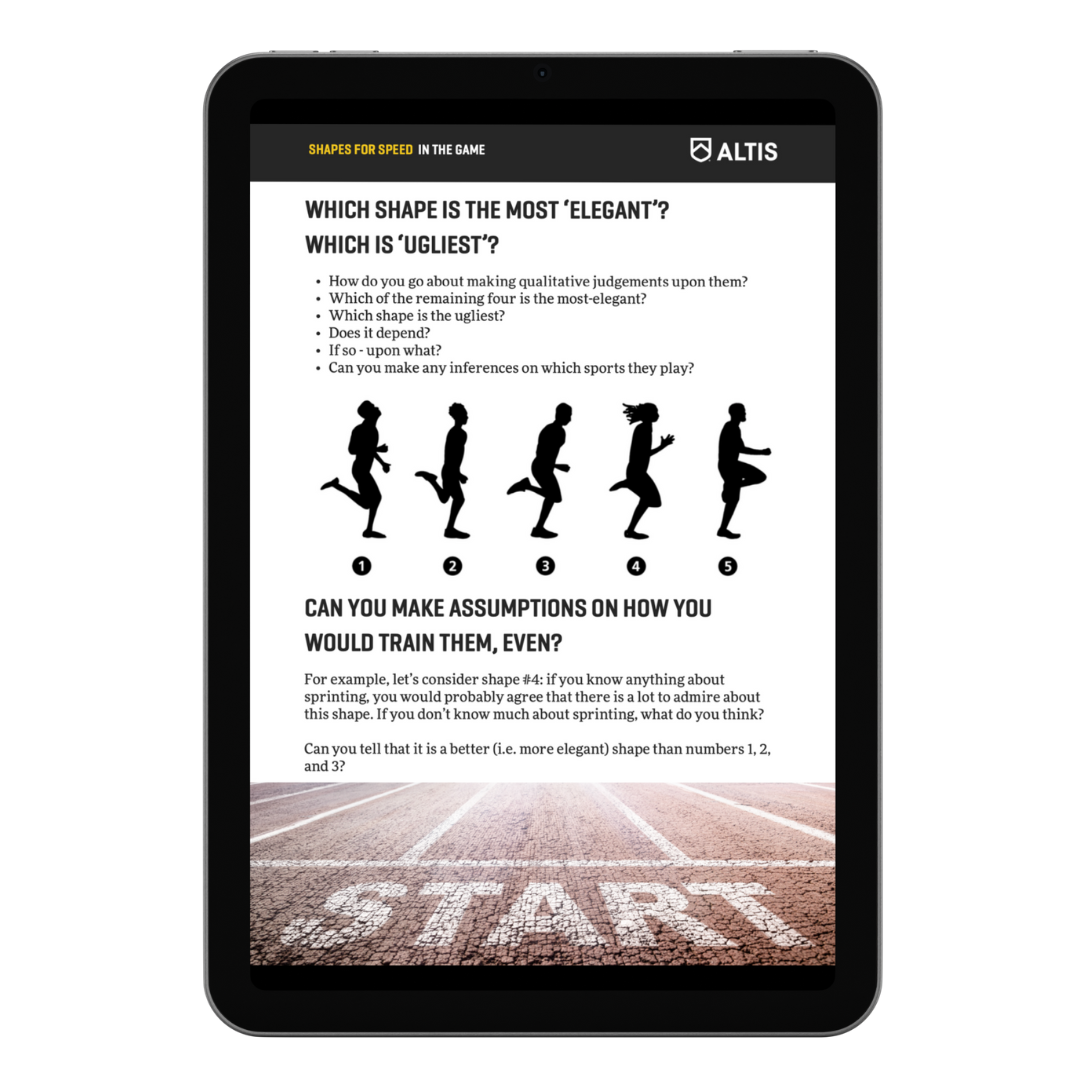