Stop Dragging your toes
Explore BLOCK CLEARANCE & EARLY ACCELERATION
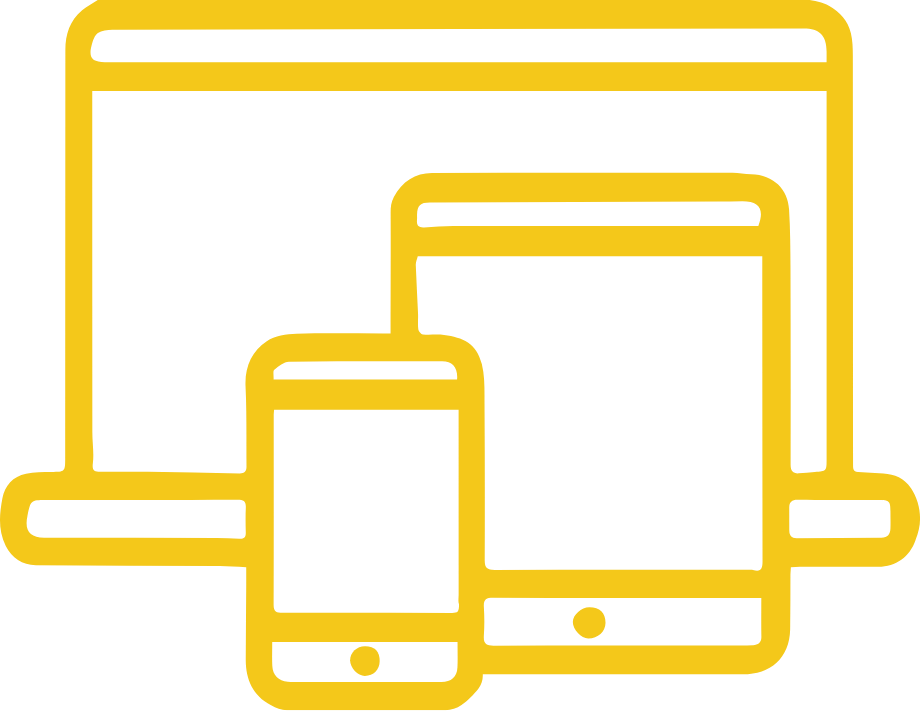
This digital course will equip you with the expertise to make informed, science based decisions about how to coach sprint starts in a way that maximizes athlete performance.
They lied to You: Dragging your toes does not improve starting efficiency...
Written by Stu McMillan, Dan Pfaff, and the ALTIS Staff, this Pocket Guide Course is a resource that provides you with everything you need to know about the specifics of the 'toe drag' concept, block clearance, and early acceleration in sprinting.
You'll learn exactly how the 'toe drag' concept came about, why proponents of this method use it, and the methodology we instead employ to get superior results - every time. Whether you coach at High School, or Elite level, 'Stop Dragging Your Toes' is the only resource you need to develop expertise on this topic, and access the most up to date information available on block clearance and early acceleration. We've done all the hard work for you, bringing the most accurate, actionable information together in one place, so you can start learning, and stop searching.
research backed content
Cut through the hype, complexity, and sea of conflicting information to access easy to understand curated content, developed by experts and proven by science.
facts, not opinions
Learn the facts about why the toe drag may be costing you valuable time and detracting from maximizing performance. Develop the expertise to coach block clearance and early acceleration with the skill of a scientist and the flair of an artist.
real world experience
Learn from the most trusted group on track - a team of Coaches who have 150+ years of combined experience, and coached 120 Olympians to 56 Olympic medals. We've done the hard work for you, so you can focus on upgrading your coaching.
Save time and effort, and get real results
Had enough of digging around on social media trying to find answers amongst a sea of conflicting information?
We've got you: This course provides all the answers you need on how to coach block clearance and early acceleration, why we coach it using specific methods and frameworks,and what is really important.
If you want to develop expertise on this topic, there is no better resource out there to equip you with the tools you need to get real results.
Everything you need to know. Nothing you don't.
This pocket guide course is packed full of information, context, case studies, videos, experiential learning, and science-backed evidence. You'll learn everything you need to know to make informed, effective coaching decisions.
Why 'Pocket Guide?' ... This exciting new course is part of our new 'Pocket Guide' series featuring deep dives into specific topics that you can complete in just a day or two.
A selection of the core themes covered are outlined below. Scroll down the page for the full course content.
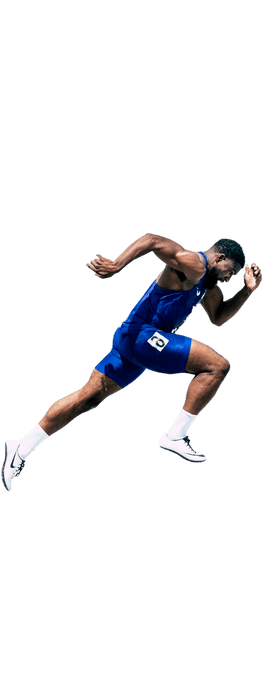
WHY WE DON'T OBSESS ABOUT HEEL RECOVERY HEIGHT
There is continued debate in the track and field world around the recovery height of the heels during early acceleration in sprinting. Here at ALTIS, we actually don't obsess over 'low recovery of heel' in the initial steps. Learn why in this course.
HOW WE ANALYZE MOVEMENT
To effectively coach the sprint start, you need to understand how to analyze movement in the first place. This course will teach you the key steps to being able to do this.
HOW TO ADDRESS TECHNICAL CHANGE
You'll be introduced to the framework through which we go about making technical changes with the athletes we work with - and thus the decision-making process of how to affect block clearance and initial acceleration.
WHERE THE TOE DRAG CONCEPT CAME FROM
It is important to understand that the genesis of this particular technique was as an error-correction reaction for athletes who would accelerate with an exaggerated cyclical heel recovery. We explain why copying this blindly is an error.
THE IMPACT OF FOOTWEAR
Differences in footwear and running surface are the two most-significant environmental constraints that will affect early acceleration technique. Learn how footwear and surfaces will change what you see in terms of early acceleration.
WHY "YOU CAN'T ROB PETER TO PAY PAUL"
You'll get to understand how the impact of mechanical changes in the start and early acceleration impact the rest of the race, and why all changes should be viewed as part of a whole.
Become the go-to Coach for the sprint start
Armed with answers and understanding, you'll finally see what's really important when it comes to the sprint start. You'll also learn why the toe-drag isn't for everyone, and how it detracts from performance - despite its well-intentioned use by some. While the start isn't the only important element of successful sprint performance, maximum step frequency is reached by the 3rd or 4th step. If you don't set this up correctly, you'll pay the price later on in the race. Can you afford not to coach this part of the race with laser accuracy?
Course Content
Packed with coaching insights, videos, anecdotes, graphics, case studies, and facts, Stop Dragging Your Toes is made up of seven carefully crafted core modules that you can navigate on your own terms, in just a day of focused study, or at a pace that suits you.
INTRODUCTION
We talk through how to navigate and get the best out of the course, set you up with access to the ALTIS Community, and share a welcome message from Coach Stuart McMillan.
MODULE I
You'll learn where the toe drag concept came from and why it isn't all it's cracked up to be; what downstream mechanical issues it causes; plus the impact of footwear on starting mechanics.
MODULE II
In this module, we start with asking the problem 'why?' before exploring the solution via our framework for coaching the sprint start, and how we go about addressing technical change.
MODULE III
You'll explore the purpose of the start (and it's not what some people think it is!) The role of mechanics, and how internal and external factors impact starting effectiveness and efficiency.
MODULE IV
You'll explore the crux of the 'movement problem' we are addressing, and the 'movement solution' we are looking for, via a 5-step framework that gives you the roadmap you need to be more effective in coaching the sprint start.
MODULE V
This module explores what we can actually do in practice to determine what steps to take, and understand how each athlete should move. This is underpinned by an exploration into individual movement and unique athlete abilities: Despite what some people say - one size does not fit all.
MODULE VI
You'll explore how block technique is placed as part of a whole race, and why it should not be viewed in isolation. You'll also learn how to reframe technique to allow you to cater to a range of athlete types and abilities to maximize individual performance. Again - one size does not fit all!
MODULE VII
This module brings everything together, and provides a tried and tested blueprint for block set up and block clearance, that you can action straight away in your environment for consistently good results.
NEXT STEPS
In this final module, you'll download your graduation certificate and get pointers and tips on recommended next steps to continue your development and upgrade your learning.
The Gold Standard in Coaching Education
BY ALTIS COACHES
BY ALTIS-COACHED ATHLETES
BY ALTIS
Frequently Asked Questions
HOW LONG WILL THIS COURSE TAKE TO COMPLETE?
This course is part of our new 'Pocket Guide' Series. These are concise, topic-specific courses that can be completed in a day of dedicated study, or a few days at a more leisurely pace. These courses provide concise, focused and actionable information that you can apply straight away to get real results.
HOW IS IT DIFFERENT FROM YOUR OTHER COURSES ON SPEED?
This course is a deep dive specifically focusing on the mechanics of block clearance, early acceleration, and the toe-drag concept in the sprint start.
It is an in-depth analysis specifically relating to developing expertise in these topics, and as such, the content is new and different to what you'll find in Coaching the Short Sprints, or Need for Speed.
If you're specifically interested in learning more on the mechanics and process of the sprint start, and how the toe-drag impacts starting, or whether or not to use the toe drag, this course is for you.
It is also an excellent bolt-on for those who are course members of Need for Speed or Coaching the Short Sprints, and are interested in upgrading their knowledge of the start and acceleration mechanics further.
CAN I REFER BACK TO IT ONCE I'VE COMPLETED IT?
Yes! As with all our courses, you get lifetime access and can dip in and out as often as you wish. You'll also automatically get access to all future course additions and updates.
CAN I ASK QUESTIONS?
Yes! If you have questions you can interact with ALTIS Staff on the ALTIS Community on Mighty Networks, which you'll get automatic access to upon course purchase. This community is a place to ask questions, and chat around themes with ALTIS Staff and motivated coaches from around the globe.
DO I GET A CERTIFICATE?
Yes! Upon course completion, you'll be prompted to download your certificate of completion, which you can print out for your files.
ARE THERE ANY CEUS WITH THIS COURSE?
No. This course is ALTIS-Accredited, but does not come with any external provider CEUs.
WHAT CAN I DO NEXT AFTER THIS COURSE?
We recommend the next step following this course is to explore 'Coaching the Short Sprints'. This 5 star reviewed course, written by Coaching Legend, Dan Pfaff - is the most comprehensive resource around on all things Short Sprints. It expands on this, and everything else you need to know to succeed as a Coach in the 100m and 200m events.
And it gets better - once you complete this course, you get a discount on Coaching the Short Sprints - win, win!
CAN I DOWNLOAD THE CONTENT?
No. This course is a digital course that you can study on any digital device with an internet connection. This allows us to make automatic updates over time and provide you with the freshest, most dynamic learning experience. You can access everything at the palm of your hand via your MY ALTIS Education Account, anytime, anywhere.

Start Totally Risk Free
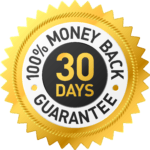
We're so confident that this course will improve your understanding and practice, that if you're not completely satisfied with your course within 30 days of purchase, you can choose from two options:
1. Full Exchange: Swap your course for another product of equal or lesser value. You'll receive a full credit towards the new offering.
2. Refund with Processing Fee: Request a cash refund. We'll process your request promptly, minus a small processing fee of 9% to cover administrative costs.